
InMemoriam
Acktually 2020cel
★★★★★
- Joined
- Feb 19, 2022
- Posts
- 10,257
Physical Time and Human Time
George Ellis1,2
October 18, 2022
George Ellis1,2
October 18, 2022
ABSTRACT. This is an invited comment on both Gruber et al (2022) and Bunamano and Rovelli (2022), which discuss the relation between physical time and human time. I claim here, contrary to many views discussed there, that there is no foundational conflict between the way physics views the passage of time and the way the mind/brain perceives it. The problem rather resides in a number of misconceptions leading to the representation of spacetime as a timeless Block Universe. The physical expanding universe is in fact an Evolving Block Universe with a time-dependent future boundary. This establishes a global direction of time that determines local arrows of time. Furthermore time passes when quantum wave function collapse takes place; during this process, information is lost. The mind/brain acts as an imperfect clock, which coarse-grains the physical passage of time along a world line to determine the experienced passage of time, because neuronal processes take time to occur. This happens in a contextual way, so experienced time is not linearly related to physical time in general.
1. Introduction: The Issues
The paper “Physical Time Within Human Time” (Gruber Block and Montemayor 2022, hereafter GBM) is in effect a response to “Bridging the neuroscience and physics of time” (Bunamano and Rovelli 2022, hereafter BR). It gives a survey of differing views of the nature of time, in particular quoting claims by some physicists and philosophers that the perceived passage of time (the Flow of time, or FOT) is an illusion because we live in a timeless block universe. In that case, there is a lack of a passage of time when viewed from a physics viewpoint, but a dominance of the passage of time in experienced consciousness. This results in a “two times problem”: the relation of veridical time (physical time), which does not pass, and manifest time (psychologically experienced but illusory time) is problematic.
BR raise two physics issues underlying the supposed problem:
T1) Relativity is incompatible with an objective notion of a global present—for example, it does not make sense to ask what an astronaut on a distant spaceship is doing “now”. This has to be resolved by having a Block Universe, where the “present” does not exist.
T2) All elementary mechanical laws of nature that we know are invariant under reversal of the direction of time—a principle referred to as charge conjugation, parity inversion, time reversal (CPT). These include classical mechanics, electrodynamics, quantum theory, general relativity, quantum field theory, and the standard model. They exhibit no arrow of time. However emergent properties do.
A solution is proposed by GBM based in the idea (Hartle 2005, 2014) of an IGUS (Information Gathering and Using System) as the basis of manifest time within the context of a block universe. Such systems arise through natural selection which begets the illusory system for functional purposes.
My overall response is given in Ellis (2014), Ellis and Goswami (2014), and Ellis and Drossel (2020): we live in an Evolving Block Universe (EBU) which is not a static spacetime block extending to future infinity, but rather a spacetime with a time-dependent future boundary that continually extends to the future and thereby establishes a global direction of time. The local arrows of time such as those underlying the possibility of evolution and brain processes derive from this direction of time in the EBU. The problems T1) and T2) are both resolved by the basic feature that emergence of complexity is always accompanied by symmetry breaking (Anderson 1972).
Mathematics Department, University of Cape Town. email: [email protected]
The New Institute, Hamburg. email: [email protected]
1
2. The Block Universe idea is based on a number of misconceptions
The block universe model does not describe the real universe (Section 2.1), because of broken symmetries: both Lorentz symmetry and general covariance are broken in the real universe (Section 2.2). Also a maximally extended spacetime does not describe the universe today: it is a manifold with finite past and future boundaries (Section2.3).
2.1 A Block universe or an evolving block universe? Contrasting views
The context for considering the issue is the present day understanding of the expanding universe (Peter and Uzan 2013, Ellis and Uzan 2014), as summarized in the famous NASA picture (Figure 1). NASA thinks the Universe has an age: indeed Aghanim et al (2020) report the age of the universe to be 13:8 Gyr.
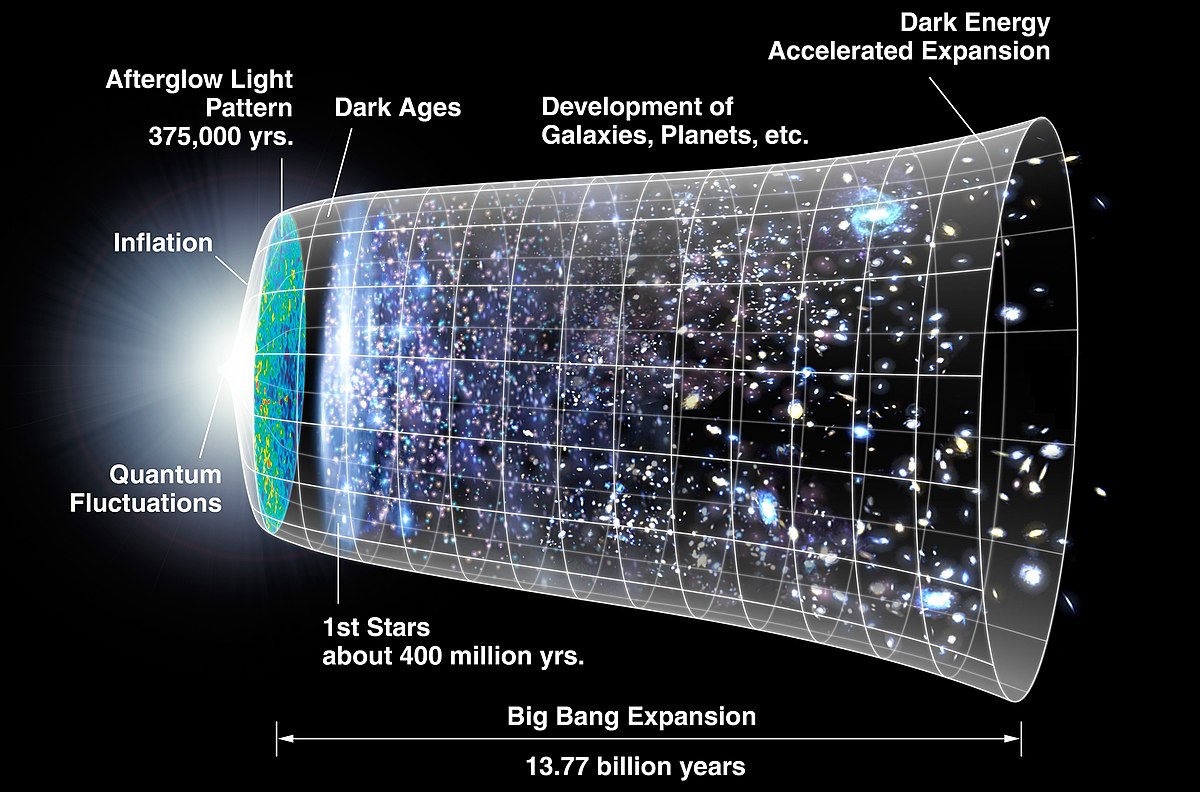
Figure 1: The expansion of the universe. In this diagram, time passes from left to right, so at any given
time, the universe is represented by a disk-shaped "slice" of the diagram (Source: Wikimedia Commons)
Those promoting the Block Universe idea think it does not have an age. Again, working cosmologists believe primordial nucleosynthesis started when the universe was 200 seconds old, decoupling when it was a three hundred thousand years old, galaxy formation a while later, and so on. Physical events unrolled as the universe evolved and grew older. This is nothing like the view proposed by those who claim we live in a block universe. How can this hiatus be solved?
2.2 Broken symmetries as the basis of emergence of cosmology
In the real universe, symmetries are broken, as happens in all cases of emergence (Anderson 1971).
Underlying the block universe idea are two symmetry related errors :
GR, not SR. Lorentz invariance holds when Special Relativity (Einstein 1924) is a good description, but does not hold when gravity is significant, as in cosmology, which is described by General Relativity (GR). The metric tensor gij(xk) is determined by the matter present through the Einstein Field Equations (Hawking and Ellis 1973). It determines proper time τ along any timelike world line xi(v):
dτ2 = - gij(xk) dxi dxj . | (1) |
This time is measured by a clock (an ideal oscillator) travelling on that worldline. .
2
B) Not GR in general, but specific solutions. General covariance is foundational to general relativity in general (Einstein 1924, Hawking and Ellis 1973), but does not hold in specific cases, where symmetries are broken (Ellis and Matravers 1995). There are preferred spatial sections in the standard background cosmological models (Hawking and Ellis 1973), and preferred timelike lines and associated spatial sections in all plausible cosmological models (Ellis 2009, Ellis and Goswami 2014).
2.3 Not a maximally extended manifold M: the present time
Since the ground-breaking work of Penrose (1965), geodesically complete, hence maximally extended, spacetimes have been extensively studied (Hawking and Ellis 1973), and this leads to representation of a block universe (BR: “static eternalism”). To get a representation congruent with the cosmologist’s view as in Figure 1, we the idea of a manifold M(t) with a future boundary M+(t) that changes with time. The technical concept needed is that of manifold with boundary (White 1952, Lang 2012). The real universe is a manifold with a time dependent boundary. The past exists and the future does not, as in Figure 1. An earlier manifold M(t1) is isometrically imbeddable as a subset in the later manifold M(t2), which is therefore an extension of M(t1) (Figure 2). Earlier and later states of the universe are distinguished by the fact that the earlier states are submanifolds of the later states. This introduces a global Direction of Time, pointing from the fixed past boundary (the start of the universe) to the continually moving future boundary (the present, at any specific time).
Figure 2 The Evolving Block Universe and the Direction of Time The manifold M(t1) is the universe at an earlier time t1 , with future boundary at t = t1, and the manifold M(t2) the universe at a later time t2 > t1. The start of the universe is at t=0. The manifold M(t1) is isometrically the same as M(t2), up to the time t1; the extra domain from t1 to t2 is the amount the universe has grown between those times. The fact that M(t1) can be isometrically imbedded in M(t2) determines that the latter is a later state of the universe than the former. This establishes the global Direction of Time pointing from t1 to t2, whether or not the universe continues expanding in the future.
The past exists because it influences the present, for example via nucleosynthesis in first generation stars. If the past did not exist, we would have uncaused entities on Earth today (the elements of life). The future does not exist because its outcomes are not yet determined, because of intrinsically random quantum events (Acín and Masanes 2016), and additionally the inability to specific initial data to arbitrary accuracy (Robertson 1929, Ellis et al 2018), leading to unpredictable classical stochasticity (Del Santo and Gisin 2019, Neyrinck et al 2022).
3
3 A global direction of time and local arrows of time
The context of the expanding universe introduces a global direction of time (Section 2.3) which breaks time symmetry (Ellis and Drossel 2020). Local basic arrows of time are derived in a downward way in this context (Section 3.1). The laws are time symmetric, but their context (Figure 2) is not; that is the source of the broken symmetry leading to local arrows of time. The basic laws then determine derived arrows of time in emergent systems (Section 3.2.
3.1 The four basic arrows
Four basic local arrows of time derive from the Direction of Time in an EBU context (Ellis 2014).
The thermodynamic arrow of time needs special initial conditions (Penrose 1990, 1995, Albert 2000), and a dark night sky to act as a heat sink (Penrose 1990, Layzer 1976). The decrease of temperature with time in the expanding universe underlies processes such as nucleosynthesis, decoupling, and star formation (Peter and Uzan 2013).
The electrodynamic arrow of time is discussed in Wheeler and Feynman (1945), Gold (1962), Layzer (1976). In an EBU advanced and retarded potential are not equivalent because the future does not yet exist, so there can be no advanced potential and associated Green function.
The gravitational radiation arrow of time is resolved similarly.
Quantum physics does not preserve information because quantum measurements (wave function collapse) involve a loss of information (Ellis 2012). Real quantum measurements (Drossel and Ellis 2018) are not time symmetric because any apparatus interacts with heat baths that affect outcomes (Drossel 2017, 2020), and thereby get an arrow of time from the universe associated with information loss.
3.2 The derived arrows
The way emergence of complex systems on smaller scales took place, leading to life, is chronicled in Morowitz (2002). Layzer (1976) comments, “Information is generated whenever the expansion (contraction) rate exceeds the rate of a local equilibrium- maintaining process”. It is through these processes of emergence that the fundamental arrows of time chain up and lead to derived arrows of time in all emergent systems (Ellis and Drossel 2020):
Diffusion: stirring a liquid, density gradients leading to fluxes, and so on.
Fracture: dropping an egg or a glass.
Waves: sound waves are heard after they are emitted.
Chemical processes: the Second Law of Thermodynamics determines from among all possible
chemical changes, those that can occur spontaneously as time progresses (Atkins 2014: 28).
Biology: physiological and developmental processes follow from chemical ones, underlying the functioning of life. The word “function” only makes sense in a context where time passes.
Biology: Natural selection begets the functional systems and cannot take place if time does not
pass: selected phenotypes and genotypes exist after they are selected. This extends to evolution of the conscious brain, capable of experiencing the passage of time.
Brain function occurs as time passes. Action potential spike chains propagate down dendrites
and axons, signalling molecules diffuse across synaptic clefts, neural network strengths are altered via gene regulation, allowing learning to take place, and so on.
Lynn et al (2022) discuss the decomposition of the local arrow of time in interacting systems. There is a cascade down of the basic arrows, and a cascade up of the derived arrows (Ellis 2014). In this way, the statement “For a neuroscientist, time is oriented, always pointing towards the future” (BR) agrees with what a physical cosmologist determines.
4
4. Multiple interacting timescales in the brain, which is not an ideal clock
We agree with BM: “The brain is an inherently temporal organ because in many ways its primary function is to learn from the past in order to best predict the future”. The IGUS view (Hartle 2005, 2014) is a simplified model of the current predictive processing view of brain function (Clark 2013, Friston 2018). The spacetime view associated with it by Hartle and GBM is an unnecessary add-on: these processes underlie memory and perception in either a Block Spacetime, or an Evolving Block Universe, and do not prefer either.
The hierarchically organized brain (Raut et al 2020) is the basis of manifest time via multilevel cortical processing (Buonomano 2007) with neural networks acting as clocks (Mauk and Buonomano 2004, Karmarkar and Buonomano 2007). Timekeeping processes in the brain are due to emergent oscillatory circuits interacting at multiple timescales (Déli et al 2017). It takes time for brain processes to happen on various scales, leading to the “specious present”. This results in our experience of time: possibly the most fundamental aspect of consciousness and indeed human existence.
At very short timescales there may be experimentally created postdictive effects (GBM), but that does not matter for conscious thoughts and decisions and memory effects at the macro scale. The experience of the irreversible passage of time, at timescales of seconds and up, is a fundamental aspect of that consciousness. Insofar as any illusions about this may occur, they are due to the way various brain components interact via oscillatory circuits to generate the sense of consciousness, on the basis of standard physical and chemical reactions.
Experiential past, present, and future are not properties of four-dimensional spacetime, but notions describing how individual IGUSs, including humans, process information in an EBU.
5. Conclusion
The characterization of different aspects of time in Section 4 of BR is useful. My proposal agrees with all of them except (iii), where I disagree, as explained above in Sections 2.2 and 2.3 above. Physical time passes. It is perceived to pass by the brain: perceiving things, adding events to memory, planning what happens and then taking action, and so on, but
it is coarse grained by the brain because of the time brain processes take to complete, and their interaction at various scales
there is no reason why the brain should be a good clock: the appearance of time passing is an aspect of perception, which is a context dependent predictive processing function. GBM give many useful examples. Time may indeed appear to go slow in some circumstances and fast in others, because brain time TB and proper time τ are not linearly related (Droit-Volet and Meck 2007).
The arguments by GBM supporting an IGUS interpretation of how we perceive time to pass is fine: it fits in with current neuroscience, and can be accommodated in an EBU context.
Where I part company with them is the statement “The phenomenon of dynamism is an experimentally demonstrable illusory experience”. That is an incoherent statement. You can’t have any experience whatever if the passage of time is an illusion. Consciousness is real (Pigliucci 2019) and the experienced passage of time is a key part of the package (Edelman 2001), whether it accurately represents physical time or not. You can have misleading experiences of the passage of physical time due to the contextual nature of perception, as GBM illustrate, but this all takes place in a context where conscious experience is a temporal phenomenon allowed by brain operations. These are based in local physics and chemistry where time passes with an arrow of time inherited from cosmology.
Similarly, I do not agree that the “persisting self” is an illusion. The body persists because of physical conservation laws; memories are made physical through brain plasticity (Kandel 2001). These memories
5
can be retrieved and constitute the core of the mental persistent self: one of the basic features of consciousness.
It is indisputable that time passes in our experience (you could not be reading this text if that were not so). This is crucial evidence as to the way things work. There is no discordance with physics if we adopt the view put in this paper.
References
Acín, A, and Masanes, L (2016). "Certified randomness in quantum physics." Nature 540: 213-219. Aghanim, N., Akrami, Y., Ashdown, M., Aumont, J., Baccigalupi, C., Ballardini, M., Banday, A.J., Barreiro, R.B.,
Bartolo, N., Basak, S. and Battye, R., (2020). Planck 2018 results-VI. Cosmological parameters. Astronomy and Astrophysics 641:A6.
Albert, D (2000) Time and Chance (Harvard Uiversity Press)
Anderson, P. W. (1972). “More is different: broken symmetry and the nature of the hierarchical structure of science”. Science, 177(4047), 393-396.
Atkins, P (2014) Physical Chemistry: A Very Short Introduction (Oxford: Oxford University Press) Buonomano DV (2007) The biology of time across different scales. Nat Chem Biol 3:594-597. Buonomano, D, and Rovelli, C (2022) Bridging the neuroscience and physics of time arXiv preprint
arXiv:2110.01976.
Clark, A. (2013). Whatever next? Predictive brains, situated agents, and the future of cognitive science. Behavioral and brain sciences, 36(3), 181-204.
Del Santo, F., and Gisin, N. (2019). Physics without determinism: Alternative interpretations of classical physics. Physical Review A, 100(6), 062107.
Déli, E., Tozzi, A., and Peters, J. F. (2017). Relationships between short and fast brain timescales. Cognitive neurodynamics, 11(6), 539-552..
Droit-Volet, S., and Meck, W. H. (2007). How emotions colour our perception of time. Trends in cognitive sciences, 11(12), 504-513.
Drossel, B. (2017). Ten reasons why a thermalized system cannot be described by a many-particle wave function. Studies in History and Philosophy of Science Part B: Studies in History and Philosophy of Modern Physics, 58, 12-21.
Drossel, B. (2020). What condensed matter physics and statistical physics teach us about the limits of unitary time evolution. Quantum Studies: Mathematics and Foundations, 7(2), 217-231.
Drossel, B., and Ellis, G. (2018). Contextual wavefunction collapse: An integrated theory of quantum measurement. New Journal of Physics, 20(11), 113025.
Edelman, G. (2001). Consciousness: the remembered present. Annals of the New York Academy of Sciences, 929(1), 111-122.
Einstein, A. (1924). Relativity: the Special and the General Theory. Trans. R. W. Lawson. (London:
Methuen).
Ellis, G (2009) Republication of: Relativistic cosmology General Relativity and Gravitation 41: 581–660.
Ellis, G. F. (2012). On the limits of quantum theory: Contextuality and the quantum–classical cut. Annals of Physics, 327(7), 1890-1932.
Ellis, G. (2014). The evolving block universe and the meshing together of times. Ann. N. Y. Acad. Sci. 1326,
26–41.
Ellis, G., and Drossel, B. (2020). Emergence of time. Foundations of Physics, 50(3), 161-190. Ellis, G. F., and Goswami, R. (2014). Spacetime and the passage of time. In Springer handbook of
spacetime (pp. 243-264). Springer, Berlin, Heidelberg.
Ellis, G. F. R., and Matravers, D. R. (1995). General covariance in general relativity?. General Relativity and Gravitation, 27(7), 777-788.
Ellis, G. F., Meissner, K. A., and Nicolai, H. (2018). The physics of infinity. Nature Physics, 14:770-772.
6
Ellis, G F, and Uzan, J-P (2017) Modern Cosmology, Scholarpedia, 12(8):32352.
Friston, K. (2018). Does predictive coding have a future?. Nature neuroscience, 21(8), 1019-1021.
Gold, T (1962) “The Arrow of Time” American Journal of Physics 30:403.
Gruber, R. P., Block, R. A., and Montemayor, C. (2022). “Physical Time Within Human Time,” Frontiers in Psychology (Hypothesis And Theory). doi: 10.3389/fpsyg.2022.718505
Hartle, J. B. (2005). The physics of now. Am. J. Phys. 73: 101–109.
Hartle, J. B. (2014). Classical and quantum framing of the Now. Phys. Today 67:8.
Hawking, S. W., and Ellis, G. F. R. (1973). The Large Scale Structure of Space-time (Cambridge University Press).
Kandel, E R (2001) ``The molecular biology of memory storage: a dialogue between genes and
synapses'' Science:294:1030-1038
Karmarkar, U. R., and Buonomano, D. V. (2007). Timing in the absence of clocks: encoding time in neural network states. Neuron, 53(3), 427-438.
Lang, S. (2012). Differential and Riemannian manifolds (Springer Science & Business Media).
Layzer, D. (1976). The arrow of time. The Astrophysical Journal, 206, 559-569.
Lynn, C. W., Holmes, C. M., Bialek, W., and Schwab, D. J. (2022). Decomposing the local arrow of time in interacting systems. Physical Review Letters, 129(11), 118101.
Mauk, M. D., & Buonomano, D. V. (2004). The neural basis of temporal processing. Annual review of neuroscience, 27(1), 307-340.
Morowitz, H (2002) The Emergence of Everything: How the World Became Complex (Oxford University Press)
Neyrinck, M., Genel, S., and Stücker, J. (2022). Boundaries of chaos and determinism in the cosmos.
arXiv preprint arXiv:2206.10666.
Penrose, R. (1965) Gravitational collapse and space-time singularities. Phys Rev Lett 14:57.
Penrose, R (1990) The Emperor's New Mind, Oxford U.P., New York ch. 7.
Penrose, R (2010) Cycles of Time: An Extraordinary New View of the Universe (Random House) Peter, P and Uzan, J-P (2013). Primordial Cosmology (Oxford Graduate Texts). Pigliucci, M (2019) Consciousness is real Aeon 16 December 2019
Raut, R. V., Snyder, A. Z., & Raichle, M. E. (2020). Hierarchical dynamics as a macroscopic organizing principle of the human brain. Proceedings of the National Academy of Sciences 117: 20890-20897.
Robertson, H. P. (1929), "The Uncertainty Principle", Phys. Rev., 34: 163–64.
Wheeler, J. A., and Feynman, R. P. (1945). Interaction with the absorber as the mechanism of radiation. Reviews of modern physics 17:157.
White, P. A. (1952). Some characterizations of generalized manifolds with boundaries. Canadian Journal of Mathematics, 4:329-342.
2022/10/18
7